
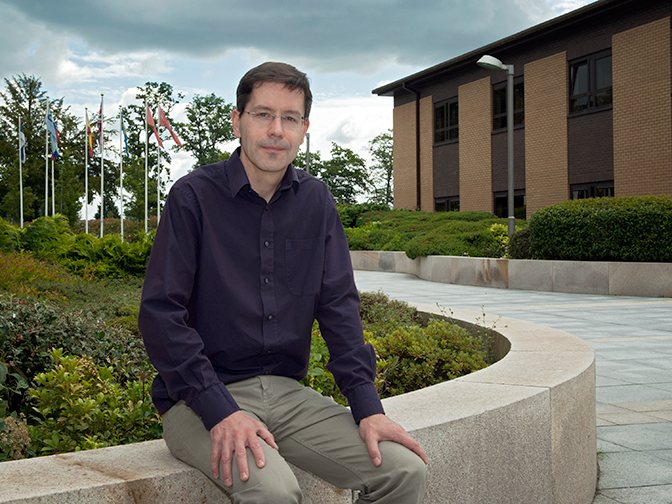
Clouds and precipitation are among the more challenging weather parameters to forecast accurately. ECMWF scientist Richard Forbes specialises in modelling the multi-scale processes involved.
Global numerical weather prediction (NWP) divides the atmosphere into millions of grid boxes whose sides are typically several kilometres long. The laws of physics can be used to predict the way in which parameters such as wind and atmospheric pressure change over time in each grid box.
But there are a number of phenomena, such as clouds, turbulent mixing and radiation, that act at much smaller scales and can be highly variable even within a single grid box. In particular, clouds and precipitation are the result of processes at the molecular scale acting on a large number of water droplets or ice crystals. All this needs to be represented in models.
Dr Forbes is one of the scientists at ECMWF who specialise in this area, which is often called the model physics.
Getting the model physics right helps to improve weather forecasts, for example to predict where and when it is going to rain. This is important not just so that we know when to carry an umbrella.
“Being able to forecast heavy or prolonged rainfall leading to flooding, or significant amounts of snowfall, can help people to prepare in advance for the impacts of severe weather,” Dr Forbes points out.
Between 1970 and 2012, storms and floods accounted for 79% of weather and climate-related disasters and caused 54% of deaths, according to the World Meteorological Organization (WMO). (Photo: SlobodanMiljevic/iStock/Thinkstock)
A physicist by training, Dr Forbes has always been interested in how the natural world works. He pursued a career in meteorology because of the impact the weather has on people’s lives.
His varied career has taken him from the UK Met Office, where he helped to develop a global ocean forecasting system, to collaboration with the University of Reading, where he worked on the prediction of extra-tropical cyclones and high-resolution models to predict convective storms. He joined the team working on physical processes in ECMWF’s Research Department in 2007.
Understanding interacting processes
One of the challenges involved in modelling the physics of the atmosphere is to adequately represent the interaction between different processes. Dr Forbes gives the example of fog, a high-impact weather phenomenon with consequences for aviation and transport, but difficult to predict.
“The formation and clearance of fog is sensitive to a number of interacting processes, such as surface characteristics, turbulence, radiation and cloud physics,” he observes. “We need to understand all the separate processes and their interactions in order to improve the forecasts.”
Another case in point is freezing rain. Its occurrence depends on the vertical temperature profile of the atmosphere and the amount of precipitation falling through it.
If a layer of warm air sandwiched between two sub-zero layers of air is sufficiently shallow, falling snow melts only partially and refreezes into ice pellets as it re-enters the near-surface cold layer (left). If the layer of warm air is deep enough, on the other hand, the snow turns into rain completely and falls as supercooled raindrops through the near-surface cold layer, resulting in freezing rain at the surface (right).
Correctly predicting freezing rain also requires an understanding of the melting and refreezing processes involved.
“Snowflakes that melt only partially in a thin layer of warm air will rapidly refreeze to ice pellets if they hit a sub-zero temperature layer near the surface,” Dr Forbes explains.
If, on the other hand, the snowflakes melt completely in a deeper layer of warm air before they enter a sub-zero layer of air nearer the surface, the resulting raindrops will tend to remain liquid until they reach the surface.
These ‘supercooled’ raindrops then freeze on impact, potentially coating power lines, plants and road surfaces in ice.
Freezing rain occurs when supercooled raindrops fall onto sub-zero temperature surfaces. (Photo: sheilades/iStock/Thinkstock)
Collaborative research
The interactions between different physical processes mean that getting just one such process wrong can lead to unexpected errors in other areas.
A recent research project aimed to track down the cause of solar radiation errors over parts of the oceans in NWP and climate models.
Dr Forbes and his colleagues found that, in the models, too little radiation is reflected in some locations because of a lack of supercooled liquid water at convective cloud tops in the cold sector of cyclones.
“The collaboration of scientists from different areas and the combination of different data sources and evaluation techniques made it possible to identify this problem in the model and to provide quantifiable information on the magnitude of the error,” Dr Forbes says.
This vertical cross section through a region of cold-air convection shows radar/lidar-derived cloud phase based on CloudSat/CALIPSO satellite data. It shows that supercooled liquid water can be present at cloud top level at temperatures below –20°C.
Observations “vital”
The observations used in the radiation error research came from a number of satellite instruments, including a lidar that provides high-resolution vertical profiles of clouds.
“Observations are absolutely vital for understanding and modelling cloud and precipitation,” Dr Forbes emphasises.
They can come from instruments on board research aircraft, laboratory studies of microphysical processes or ground-based and satellite remote sensing.
In particular, the CloudSAT and CALIPSO satellites, which were launched ten years ago, “have provided an unprecedented view of the vertical structure of clouds around the globe.”
Artist’s impression of the CALIPSO satellite. The CloudSat and CALIPSO satellites were launched in April 2006. (Image: CNES/ill./CARRIL Pierre, 2004)
“Radar and lidar are active satellite instruments: they work by sending out signals from their position in orbit and measuring the backscatter from particles in the atmosphere,” Dr Forbes explains.
“It always amazes me how much detailed information we can obtain about the Earth and its atmosphere from space, and we are still very much learning from these observations to keep improving our models and improving our forecasts of weather into the future.”